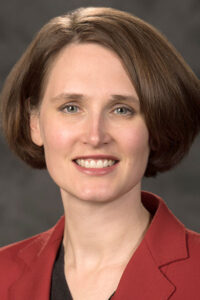
Lynette M. Sholl, MD
Lynette M. Sholl, MD, Chief of Thoracic Pathology and Associate Director of the Center for Advanced Molecular Diagnostics at Brigham and Women’s Hospital and Associate Professor at Harvard Medical School, discusses her thoughts on the “new gold standard” of a combination approach—tissue and blood-based—to testing in lung cancer. Dr. Sholl answers several questions regarding the technical applications and hurdles regarding cell-free DNA testing and RNA testing.
Q: Please discuss the use of maximum allelic frequency to improve cell-free DNA (cfDNA) testing.
A: cfDNA testing for lung cancer has come a long way since approximately 5 years ago, when we were primarily focused on point-mutation testing. The first U.S. Food and Drug Administration approval for cfDNA testing was for the EGFR T790M mutation, followed in recent years by many other approvals.
Remember that we have had blood-based diagnostics in the hematology space for a very long time; minimal residual-disease (MRD) testing has been the cornerstone of chronic myeloid leukemia management for almost 20 years. The paradigm of cfDNA testing in lung cancer really began in the resistance setting. Oncologists had to make decisions at the time of disease progression, when first-generation EGFR TKIs failed, about whether to put the patient on osimertinib or to try an alternative strategy. The easiest way to make that determination, it turned out, was a single-gene assay for T790M. Identification of that mutation predicted response to osimertinib regardless of whether it was found in the tissue or in the blood. That development has opened the door to everything that followed.
Moving from the single-gene strategy to a more comprehensive approach, laboratories were effective not only at increasing the sensitivity of sequencing techniques but also at reducing the number of errors generated by the assays. That combination of increased sensitivity and error suppression became central to our ability to move from a focused single-gene assay, with which you can get very good sensitivity but very little breadth, to a broader panel—a next-generation sequencing, DNA-based strategy—with which we could get the best of both worlds. We are obtaining good sensitivity and good breadth of coverage, and we are minimizing the technical error rate.
The challenge, however, is that this broader assay is picking up more than just the patient’s lung cancer. It is picking up incidental signals as well, such as clonal hematopoiesis variants or other clonal processes of unclear clinical significance. We almost have too much information, and there is a question of how to deal with the distractions. Clonal hematopoiesis (CH) is a major distractor, but because many of the affected genes (DNMT3A, TET2, etc) are specific to this disorder, we can readily recognize their origin. However, certain gene alterations can be harder to interpret—such as in TP3 or occasional RAS family members that may be derived from CH but that could also be derived from a solid tumor like lung cancer.
The cleanest way to overcome confusion with clonal hematopoiesis is to run a paired buffy coat analysis and subtract the variants in the buffy coat from the plasma output. Some institutions are doing that, although it is not the norm in most commercial assays right now. It is more costly, because it requires sequencing two samples for every patient rather than just one.
Q: How do you identify variants that may be coming from another source in the patient besides the white cells or the lung tumor of interest?
A: One strategy is to start pulling in more information about the nature of the cfDNA that is in the blood, beyond mutations alone. We can look at the fragmentation profiles of the cfDNA because DNA fragments reflect where nucleosomes are bound to the DNA. This informs the gene expression patterns, which in turn reflect site of origin. There is no perfect algorithm yet, but there is plenty of evidence suggesting we can infer organ of origin from within the cfDNA by applying these other nonmutation–specific analyses.
In theory, we can couple that kind of analysis with mutation-specific analyses to determine whether a sample is showing a pure lung-derived or predominantly lung-derived specimen of cfDNA and mutations within it, which would, therefore, be relevant to the patient’s disease.
Another strategy that can confirm that you’re dealing with a patient’s relevant tumor variants is to sequence the tumor first and create a tumor-informed cfDNA test. This is one of the major debates around MRD testing—do you use the tumor-informed strategy or a sensitive patient-agnostic panel next-generation sequencing assay? The tumor-informed strategy is likely to detect lower levels of circulating tumor DNA than a more generic test. But it is also more logistically complicated. I’m sure there will be lively debate about this for the next few years, with a combination of economic, practical, and scientific considerations influencing the uptake of these different strategies.
Interpreting the allele fractions really is contextual. Some of the commercial tests indicate their estimation of what the circulating tumor DNA fraction is in a plasma specimen. With that information, you can make a little more sense out of the relevance of the individual variant allele fractions reported for different genes, and you may be able to more readily infer the presence of two or more distinct populations (such as those derived from the lung tumor versus those derived from CH).
For a targetable oncogenic driver, clinical data suggest that the absolute variant allele fraction does not change the likelihood of a patient’s response to the appropriate targeted therapy. For example, if you find an EGFR L858R mutation in the patient’s blood, it has the same likelihood of predicting response to EGFR-targeted therapy whether it’s a 0.1% or a 10% variant allele fraction. However, that number is of prognostic importance. If there is a very high varying allele fraction for your oncogenic driver, that means the patient has a very high burden of disease, which may translate into a worse outcome.
Q: Is cfDNA testing equally helpful across driver mutations and patient demographics?
A: Detection of different driver mutations depends on the cfDNA test design. In particular, whether it can comprehensively detect the broad diversity of mutations that occur in many of the newly targetable alterations in lung cancer including MET, EGFR exon 20, and fusion genes. Most NGS-based strategies are likely to have good sensitivity for diverse single nucleotide variants and insertion-deletion variants, whereas multiplex PCR strategies will have more limited ability to detect all the possible driver variants. DNA-based strategies, even using NGS, are more limited in their ability to detect fusion events.
It is possible to misinterpret germline variants in the cfDNA as being related to the tumor. It could be that your germline filtration strategies are not as robust in one demographic compared to another. However, you can rely on the fact that the fraction of circulating tumor DNA is often quite low, and this usually allows you to parse out variants derived from the germline versus those derived from the tumor.
Q: Is this—or other measures that could improve cfDNA testing—helpful with resistance and/or progression for patients with EGFR mutation or other mutations?
A: If the patient has a really high disease burden, it may be hard to make that distinction. In that case, having a paired buffy coat sample can help provide a definitive germline analysis at the same time. Could the T790M mutation in the blood be germline, or could it be somatic? We can usually infer the origin based on the variant allele fraction. But again, if tumor burden is very high, we may not be able to tell. In a pre-treatment setting, it makes sense to do a dedicated germline test. In the pre-treatment setting, an EGFR T790M mutation has about a 50-50 chance of being of germline origin. Confirming a germline variant will be important from the standpoint of genetic counseling both for the affected individual and their family members.
Q: Is DNA enough, or do we need RNA? RET-fusions, for example, have been found in RNA coming from tissue.
A: All our current circulating tumor clinical assays are DNA-based. A subset of these DNA-based assays are designed to detect fusions. Detection of fusions from plasma using a DNA-based strategy is just as challenging as it is from tissue. A lot of those challenges relate to the nature of the regions that we need to interrogate in order to find a fusion. Most of the time we are looking for fusion break points within introns, but many of the introns that we need to interrogate are very hard to sequence because they are large and have many repetitive elements. They can have a very skewed guanine–cytosine content, so there can be biases simply in capturing the sequence to analyze.
A subset of our patients with lung cancer with a history of light or no smoking have a negative DNA-based comprehensive genomic analysis. Fusions can be missed, usually for technical reasons: maybe they were not covered by the DNA panel, or maybe they are in regions of the genome that are very hard to pick up. NTRK2 fusions are a classic example of this because of the size of the introns you have to target and other challenges in obtaining good-quality sequencing in those regions. These patients benefit from a second, RNA-based fusion detection assay.
To conduct an RNA test of tumor tissue, you have to do an isolation for DNA and then a separate isolation for RNA. You can also perform a total nucleic acid isolation to divide those, and then run them two different ways. Running two different tests for DNA and RNA can be cost-prohibitive. Some labs have the means to do both upfront, but many labs do it in a sequential fashion, and only run the RNA test if the DNA test does not produce what is needed.
There is a different set of problems in the blood. There may be a historic bias—a mantra that we can’t find RNA in the blood because it is going to degrade too quickly. In fact, you can get RNA from the blood, and you may not even need a dedicated exosomal fractionation but can just perform a whole-plasma extraction. Proof-of-principle studies have indeed confirmed that you can obtain RNA sequencing from the blood. However, there is a lot of “irrelevant” RNA; that is, free RNA that is derived from blood cells. The technical challenge has been finding the needle in the haystack, the tumor transcriptome that is detectable within the blood. This challenge can be overcome using bioinformatic tools to subtract out all the signal coming from the normal cells and enhance the signal from the tumor RNA.
It is comparable to dealing with DNA, but the magnitude of the masking of the tumor’s signal is even greater. Some groups have made headway on this type of technical hurdle.1 We still need to see how reliable fusion calling from the blood will be. We will also need modifications to assays for fusion detection—specifically in the blood, because the sequences of RNA are very short, potentially even shorter than in tissue.
We have not yet seen widespread adoption of cfRNA testing yet, and there may be a number of other technical and bioinformatic hurdles to overcome. But it is possible that we will be able to get more global expression data, in addition to information on specific fusion events or oncogenic events, from RNA in the plasma.
Q: How do you respond to patients who ask for RNA testing after DNA testing comes back inconclusive?
A: We have to think about how to produce a reliable result. If a patient has had plasma testing that is negative, then they need tissue testing. After you have a negative tissue-testing result with a high-quality, informative sample, then you should move on to RNA-based testing to confirm that an actionable fusion has not been missed.
We are not at a point yet with plasma assays where we can go from a DNA plasma test to an RNA plasma test. I also don’t think you can call either blood or tissue the gold standard; I think a robust combination of the two is our new gold standard. There are plenty of situations in which the tissue alone does not generate any information at all.
There have been a couple of studies that have looked at the informativeness of a tissue specimen versus the informativeness of plasma specimen, and the numbers are strikingly similar.2,3 In about 10% to 15% of cases that are tissue negative, the plasma test finds the answer, and vice versa.
It’s hard to definitively argue right now that every patient should be getting both tests. If you have a great tissue specimen in hand, then just test it. Conversely, if you only have a plasma test but it has a clear oncogenic driver, then you have informative data. You don’t necessarily need to test both sample types at diagnosis. At the time of disease progression (especially on a targeted therapy), tissue sampling can detect histologic (small cell or squamous cell) transformation and can be used for mutational profiling, whereas the addition of plasma testing can more comprehensively reflect the heterogeneity of resistance mechanisms across sites of disease. Plasma testing, however, will not detect histologic transformation.
References
- 1. Larson MH, Pan W, Kim HJ, et al. A comprehensive characterization of the cell-free transcriptome reveals tissue- and subtype-specific biomarkers for cancer detection. Nat Commun. 2021;12(1):2357.
- 2. Schouten RD, Vessies DCL, Bosch LJW, et al. Clinical utility of plasma-based comprehensive molecular profiling in advanced non-small-cell lung cancer. JCO Precis Oncol. 2021;5:PO.20.00450.
- 3. Aggarwal C, Thompson JC, Black TA, et al. Clinical implications of plasma-based genotyping with the delivery of personalized therapy in metastatic non-small cell lung cancer. JAMA Oncol. 2019;5(2):173-180.