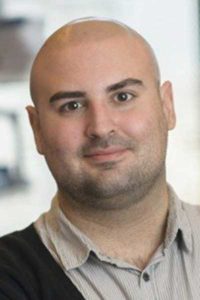
Alvaro Quintanal-Villalonga, PhD
About 70% of patients with small cell lung cancer (SCLC) present with metastatic disease at initial diagnosis. Metastasis is a major cause of morbidity and mortality in SCLC, which makes the identification of mechanisms of metastasis a priority in this tumor setting. One of the major hurdles for studying the molecular biology of metastasis in SCLC is the paucity of clinical specimens appropriate for molecular characterization.
Surgical resection of macro-metastasis is rarely performed, as chemotherapy and radiotherapy are generally effective in the initial treatment setting Thus, collected surgical specimens include almost exclusively early-stage tumors, which do not capture or reflect the complete metastatic process. As an alternative to molecular analysis of human tumor tissue, sophisticated preclinical models of metastasis have been established, including patient circulating tumor cell (CTC)-derived xenografts (CDXs)1,2 and genetically engineered mouse models (GEMMs).3 Unlike other SCLC models such as patient-derived xenografts (PDXs) or cell lines, CDXs efficiently originate metastasis in vivo.1,4
However, because CDX models are derived from cells in circulation, they are unsuitable for the study of the initial steps of metastasis (i.e. how primary tumor cells acquire the ability to escape the tumor and invade). Additionally, CDXs may not recapitulate the biology of the primary tumor cells that initially originate metastasis in patients, as CTCs can be derived not only from the primary tumor, but also from metastasis. Lastly, due to the dependence on immunodeficient mouse strains to grow them, CDXs preclude the study of interactions between the tumor and the surrounding immune microenvironment, a critical factor in the metastatic process of many different tumor types.
On the other hand, SCLC GEMMs allow molecular and functional characterization of programs driving metastatic potential in a physiological and immunocompetent context. However, tropism of metastasis in SCLC GEMMs may not fully recapitulate that of human SCLC. This is exemplified by the limited number of SCLC GEMMs exhibiting brain metastases,5 which are frequent in human SCLC. Additionally, these models harbor significantly fewer genomic alterations than human tumors,6 potentially reducing tumor immunogenicity and skewing tumor evolution, which may limit the ability of these models to recapitulate the metastatic process in humans.
In short, different limitations exist for all preclinical models available to characterize the metastatic process in SCLC.
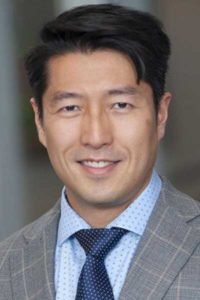
Joseph Chan, MD, PhD
To circumvent these limitations, our team has optimized techniques that facilitate single-cell RNA sequencing (scRNA-seq) in core needle biopsies, fine needle aspirates, and pleural effusions,7 thus expanding the spectrum of SCLC clinical specimens amenable for single-cell resolution analysis beyond surgical resections.
In addition, we have optimized multiplexed ion beam imaging (MIBI), a complementary imaging technology that detects multiple protein markers simultaneously at single-cell resolution. Leveraging these methods, we have constructed an integrated single-cell atlas of human SCLC samples, including primary and metastatic tumors.7
Focusing specifically on the SCLC malignant cell compartment, unsupervised clustering identified a rare cell subset representing a small portion of each tumor (ranging from 0.75-11.6% of tumor cells) (Fig. 1A). Despite the incredible heterogeneity of SCLC tumors and unlike other cell clusters identified, this subset was observed across most samples, independent of treatment history, tissue site, and SCLC subtype. Further analysis of these cells identified a potential dedifferentiated and metastatic phenotype, characterized by upregulation of genes involved in metastasis, stem-cell likeness, and by an undefined intermediate subtype state not adjusting to any of the described SCLC subtypes. Additionally, this cell cluster exhibited remarkably high expression of phospholipase C gamma 2 (PLCG2), a transmembrane enzyme involved in the production of signaling molecules, including diacylglycerol, and related to a receptor family member previously involved in metastasis (PLCG1).
Within the cluster, PLCG2 expression itself was associated with upregulation of stemness and pro-metastatic genes, nominating this gene as a driver of metastasis in SCLC. In support of this hypothesis, PLCG2 expression was higher in local and distant metastasis samples compared to primary tumors, and induced PLCG2 overexpression in isogenic preclinical SCLC models increased migration and invasion in vitro, as well as metastatic colonization in vivo.
Most remarkably, increased representation of this high PLCG2-expressing cluster predicted dramatically lower overall survival in patients (Fig. 1B). Leveraging MIBI in a tumor microarray containing an independent cohort of clinical specimens, we confirmed the existence of this PLCG2-expressing SCLC cluster (Fig. 1C) and found that tumors with at least 7% PLCG2 tumor positivity predicted worse overall survival.
Together, these results suggest that this intratumoral cell population, even if small, may have a role in SCLC metastasis driven at least partially by PLCG2, and a strong impact on SCLC prognosis.
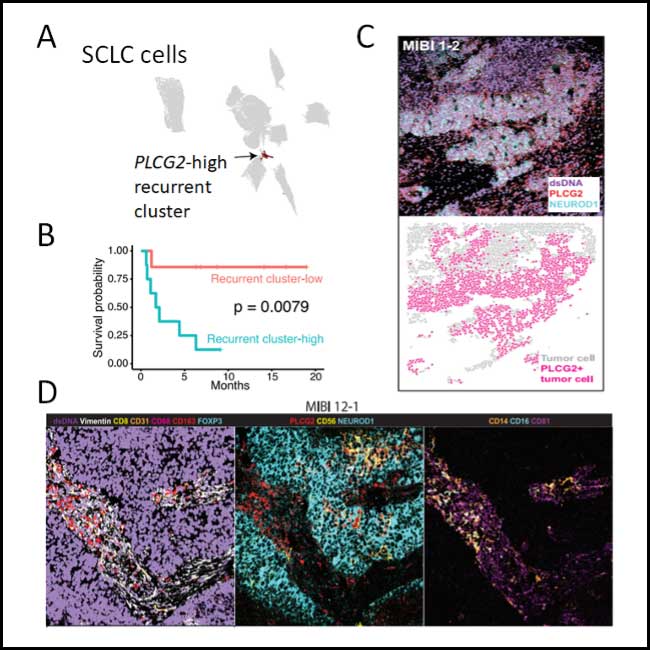
Fig. 1. The recurrent PLCG2-high cluster. (A) Uniform Manifold Approximation and Projection (UMAP) plot of small cell lung cancer cells with recurrent cluster 22 colored in black. This non-linear plot performs dimensionality reduction such that each point is a cell, and cells that are closer together have similar transcriptional profiles. (B) Kaplan-Meier analysis of overall survival in patients with detectable PLCG2+ recurrent cluster cells by scRNA-seq (≥0.75% vs. <0.75% of SCLC cells). (C) MIBI image depicting PLCG2 (red), NEUROD1 (cyan), and dsDNA (violet) channels in an example SCLC tumor, illustrating a high fraction of PLCG2-positive cancer cells (top image); and same field of view with segmented cancer cells represented by dots colored by PLCG2 positivity (bottom image). (D) MIBI images of an example SCLC tumor showing the co-occurrence of the PLCG2-positive SCLC cells and the putative profibrotic myeloid population. Left: Channels dsDNA (violet), vimentin (white), CD8 (yellow), CD31 (orange), CD68 (red), CD163 (red), and FOXP3 (cyan) illustrate the global tumor environment structure. Middle: Channels PLCG2 (red), CD56 (yellow), and NEUROD1 (cyan) identify PLCG2+ tumor. Right: Channels CD14 (orange), CD16 (cyan), and CD81 (magenta) identify the profibrotic myeloid population.
An additional benefit to profiling our clinical cohort of SCLC tumors with single-cell technologies was our ability to assess SCLC metastasis in the context of the surrounding immune microenvironment. Most SCLC tumors displayed a “cold” immune phenotype, with a reduced number of immune cells largely sequestered from the rest of the tumor.
However, we identified a CD14+ CD16+ CD81+ myeloid population enriched in SCLC tumors compared to a control cohort of lung adenocarcinoma (LUAD). These myeloid cells displayed (1) a pro-fibrotic phenotype similar to macrophages found in idiopathic pulmonary fibrosis, including high expression of pro-metastatic growth factors involved in extracellular matrix deposition and remodeling; and (2) high expression of genes involved in immune suppression; collectively, this suggested a potential role for these myeloid cells in SCLC metastasis.
Interestingly, scRNA-seq analysis found that this myeloid population was associated with the presence of the PLCG2-high SCLC cluster. And consistently, MIBI independently confirmed that more than any other cell type in the surrounding milieu, CD14+ CD16+ CD81+ myeloid cells were most strongly correlated with the presence of PLCG2+ cancer cells (Fig. 1D). These findings raise the possibility of a tumor-immune coordination in facilitating metastasis, possibly through extracellular matrix deposition by profibrotic myeloid cells. Future studies will be needed to confirm this hypothesis.
These results raise several important questions: What are the mechanisms behind PLCG2 driving metastasis in SCLC? Are there other functionally important genes in this recurrent phenotype? Is this a mechanism of metastasis specific to SCLC or might it be operative in other tumor types?
Collectively, despite high SCLC heterogeneity, this work identifies a small intratumoral cell cluster that recurs in SCLC tumors independent of tissue location, treatment, or SCLC subtype; it is characterized by high PLCG2 expression, with a potential role in the development of SCLC metastasis and a strong impact on patient outcome. We also identified a subpopulation of myeloid cells associated with this recurrent cluster exhibiting a pro-metastatic phenotype, suggesting an interplay between the tumor and the surrounding immune microenvironment that may possibly facilitate SCLC metastasis (Fig. 2).
Beyond the focus of this article on metastasis, the single-cell atlas of SCLC patient tumors also offers strategies for probabilistically classifying canonical SCLC subtypes, measuring increased phenotypic heterogeneity in SCLC compared to lung adenocarcinoma, and characterizing subtype-specific, functional differences in the T-cell compartment.
Collectively, these findings exemplify the power of multi-modal single-cell technologies. Here, we have provided both wet lab and computational tools to unravel important and clinically relevant biology in complex human tumors, including rarely resected malignancies and longitudinal small sample collections.
Raw and processed data of both scRNA-seq and MIBI from the SCLC single-cell atlas is available for exploration and download at the Human Tumor Atlas Network (HTAN) data portal. Processed MIBI data is also available for viewing at Ionpath.
References
- 1. Simpson, K. L. et al. A biobank of small cell lung cancer CDX models elucidates inter- and intratumoral phenotypic heterogeneity. Nat. Cancer 1, 437–451 (2020).
- 2. Drapkin, B. J. et al. Genomic and functional fidelity of small cell lung cancer patient-derived xenografts. Cancer Discov. 8, 600–615 (2018).
- 3. Ko, J., Winslow, M. M. & Sage, J. Mechanisms of small cell lung cancer metastasis. EMBO Mol. Med. 13, (2021).
- 4. Stewart, C. A. et al. Single-cell analyses reveal increased intratumoral heterogeneity after the onset of therapy resistance in small-cell lung cancer. Nat. Cancer 1, 423–436 (2020).
- 5. Meuwissen, R. et al. Induction of small cell lung cancer by somatic inactivation of both Trp53 and Rb1 in a conditional mouse model. Cancer Cell 4, 181–189 (2003).
- 6. McFadden, D. G. et al. Genetic and clonal dissection of murine small cell lung carcinoma progression by genome sequencing. Cell 156, 1298–1311 (2014).
- 7. Chan, J. M. et al. Signatures of plasticity, metastasis, and immunosuppression in an atlas of human small cell lung cancer. Cancer Cell 39, 1479-1496.e18 (2021).